Professor Gregory Warr is a Professor in the School of Chemistry. His research is aimed at understanding the behaviour of amphiphilic compounds in bulk phases (solutions, liquid crystals, complex fluids), in colloidal systems (emulsions, foams, dispersions), and at interfaces.
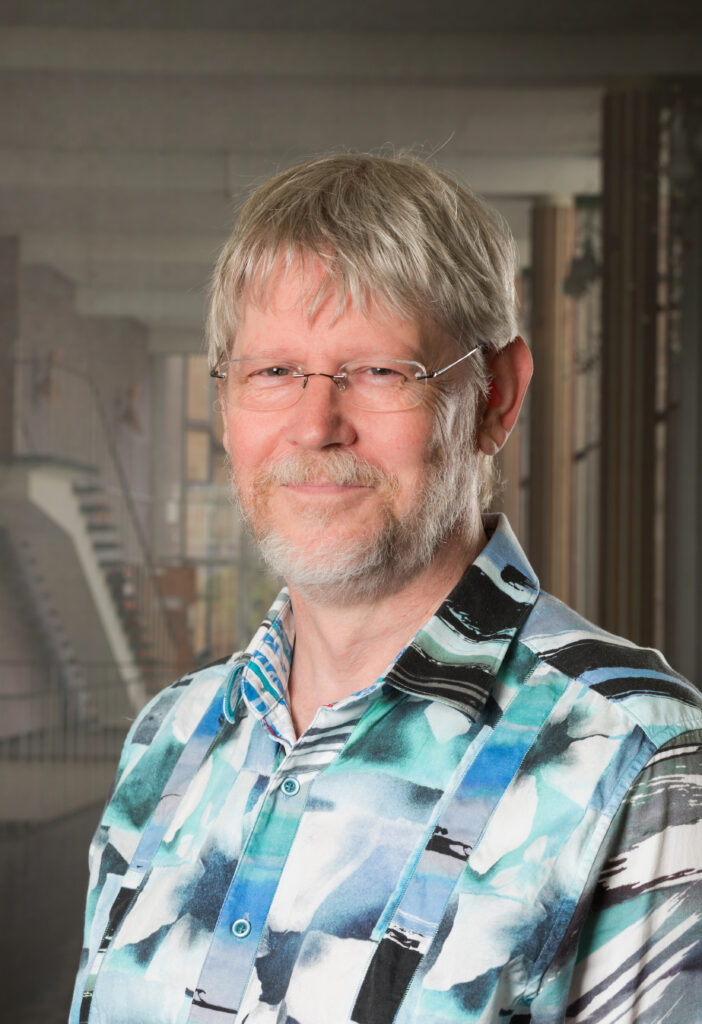
Using Big Science To See Nanoscale Structure
If you ask someone about Big Science, their mind will generally turn to particle physics, exemplified by CERN, or to astronomy, such as the Hubble or James Webb telescopes (or Laurie Anderson if you’re the right vintage). In Australia, one might think of the Square Kilometer Array radiotelescopes.
If you ask a politician why we have a nuclear reactor, they will most likely (correctly) say “to make important materials for medicine.”
Somewhat lost between these answers are two Australian Big Science facilities, both operated by ANSTO. In addition to the synthesis of medically important radionuclides like technetium, the Opal reactor at Lucas Heights produces an excess of neutrons; These are guided out of the reactor to be used for fifteen specialised beamline instruments. The Australian Synchrotron in Clayton produces a spectrum of X-rays for a comparable and complementary suite of instruments. Both facilities are used by hundreds of scientists every year to investigate the properties of thousands of soft and hard materials on the molecular and nanoscale.
Before these facilities opened in 2007, much of my research into the structure of self-assembled nanomaterials required me or my students to travel to neutron and X-ray facilities in the US, UK, France, Germany, Japan, or South Korea, to ship samples and sometimes sample environments ahead, to collaborate with local scientists, or to put things in our suitcases (and hope to not encounter an over-zealous customs officer). While this teaches you to plan carefully for all contingencies, it also constrains the kinds of experiments you can do, and what you can achieve. Today, having these major research facilities in our backyard enable us to use state of the art tools in a range of sample environments to unravel the structure and dynamics of delicate molecular assemblies such as lipid bilayers, polymer gels, emulsions, and liquid crystals on scales too small for resolution by light, and under a variety of conditions of temperature, pressure, flow, fields, and in or out of equilibrium.
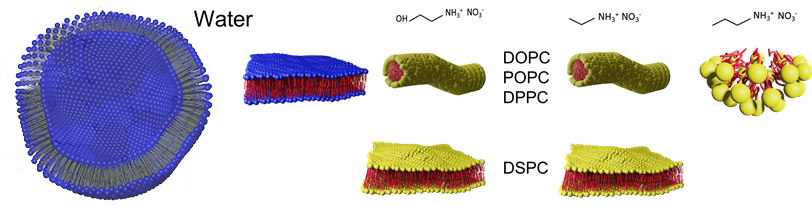
The range of problems that can be investigated using these instruments is enormous, and is attractive to international research partners as well as to industry. Together with collaborators from Tokyo University of Science, we showed how UV-visible photoisomerisation in the beamline can change micelle shape and reversibly switch a liquid between high and low viscosity.1 In a Linkage Project with Dyno Nobel we used a magnetic field to line up and squeeze ammonium nitrate droplets together to help understand emulsion stability, using neutrons to follow droplet separation and deformation.2 With collaborators from Ca’ Foscari University of Venice, we investigated whether lipid-like bilayers could form in the environment of the liquid ethane lakes of Saturn’s moon, Titan. Most recently, with collaborators from Princeton, we have used neutron and x-ray beamlines to understand how to control the structure of lipid nanoparticles used to deliver mRNA vaccines for COVID, to improve their formulation and stability for many therapeutic applications. Soon, with collaborators from City University of Hong Kong, we will look at how to improve the design of high-reflectivity paint coatings by literally watching paint dry on the nanoscale in a neutron beamline.
My main research direction over the past almost 20 years has been understanding ionic liquids – salts that are molten near room temperature – as well as solutions and self-assembled nanomaterials in ionic liquid solvents. Ionic liquids are highly polar, and share several key properties with water. We have found that they, too, can sustain globular or thread-like micelles, microemulsions, liquid crystals, and even phospholipid bilayers, but that bilayer stability is sensitive to small changes in ionic liquid composition.3-4
Ionic liquids often contain organic cations ions such as ammonium, phosphonium, or imidazolium. Recently we have moved towards more complex but biocompatible alternatives containing a choline cation and carboxylate and amino acid anions. As neutrons are particularly sensitive to hydrogen and deuterium, they are especially useful for studying organic materials. By selectively labelling one or more components of complex mixtures – polymer, lipid, ions or functional groups in the ionic liquid itself – we can isolate the role of each in the nanostructure, and understand how they influence material properties.
Armed with a sound and but still growing understanding of the fundamentals of these novel liquids, we are now examining how mixing molecular solvents can lower the viscosity and improve conductivity of ionic liquid electrolytes without reducing their effectiveness at electrode surfaces.5 Using our understanding of self-assembly, we are also designing nanostructured ionic liquid lubricants to replace toxic and persistent perfluorinated chemicals in current formulations. And, in a slightly longer-term project, we are exploring whether ionic liquids could be base solvents for a completely alien form of life: a water-free exobiology.6 At this stage we’re still using neutrons to understand self-assembled compartments much less complex than the simplest cell, so we’re not yet ready to recommend pointing the James Webb telescope at exoplanets that might be homes to ionic liquid oceans.
- 1. Akamatsu, M.; FitzGerald, P. A.; Shiina, M.; Misono, T.; Tsuchiya, K.; Sakai, K.; Abe, M.; Warr, G. G.; Sakai, H., Micelle Structure in a Photoresponsive Surfactant with and without Solubilized Ethylbenzene from Small-Angle Neutron Scattering. J. Phys. Chem. B 2015, 119 (18), 5904-5910. (10.1021/acs.jpcb.5b00499)
- 2. Jain, N.; Liu, C. K.; Hawkett, B. S.; Warr, G. G.; Hamilton, W. A., Application of small-angle neutron scattering to the study of forces between magnetically chained monodisperse ferrofluid emulsion droplets. J. Appl. Crystallogr. 2014, 47, 41-52. (10.1107/S1600576713030045)
- 3. Salvati Manni, L.; Fong, W.-K.; Wood, K.; Kirby, N.; Seibt, S.; Atkin, R.; Warr, G. G., H-bond network, interfacial tension and chain melting temperature govern phospholipid self-assembly in ionic liquids. J. Colloid Interface Sci. 2024, 657, 320-326.( 10.1016/j.jcis.2023.11.158)
- 4. Miao, S.; Hoffmann, I.; Gradzielski, M.; Warr, G. G., Lipid Membrane Flexibility in Protic Ionic Liquids. The Journal of Physical Chemistry Letters 2022, 13 (23), 5240-5245.( 10.1021/acs.jpclett.2c00980)
- 5. Wang, J.; Buzolic, J. J.; Mullen, J. W.; Fitzgerald, P. A.; Aman, Z. M.; Forsyth, M.; Li, H.; Silvester, D. S.; Warr, G. G.; Atkin, R., Nanostructure of Locally Concentrated Ionic Liquids in the Bulk and at Graphite and Gold Electrodes. ACS Nano 2023, 17 (21), 21567-21584.( 10.1021/acsnano.3c06609)
- 6. Bryant, S. J.; Garcia, A.; Clarke, R. J.; Warr, G. G., Selective ion transport across a lipid bilayer in a protic ionic liquid. Soft Matter 2021, 17 (10), 2688-2694. (10.1039/D0SM02225J)